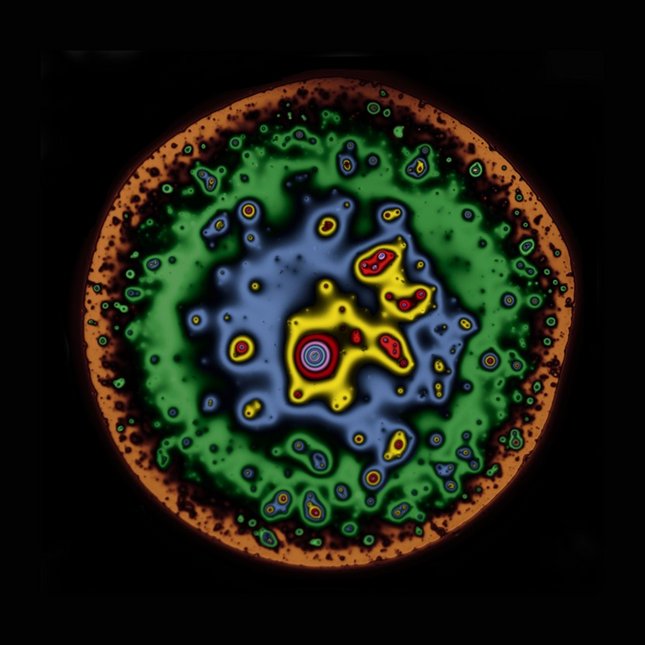
Soft matter exists in abundance in nature and inspire numerous industrial applications. In the Processing and Performance group, soft matter technology is approached experimentally, theoretically, and numerically. Soft matter covers a wide spectrum of materials and hierarchical microstructures; our group’s work reflects that by characterizing soft matter from microscopic-to-macroscopic length scales. Our group leverages this knowledge in emerging technologies such as food printing and 4D-printing.
Thermal fluctuations
Intrinsic to soft matter is that the strength of the interactions is comparable to the thermal energy kBT. This also implies that the thermal fluctuations can playa key role in the behavior of soft matter. The microscopic constituents of soft matter (e.g., small suspended particles or droplets, coarse-grained beads in a polymer) experience Brownian motion. While thermal fluctuations are most often not visible on the macroscopic scale because they are averaged out, one of our research directions is concerned with situations in which thermal fluctuations do come forward as a significant contribution, e.g., in microrheology,micro-/nanofluidics, filaments, and thin films. Efforts in this direction are currently focused on method development and numerical simulations in relation to fluctuating viscoelasticity.
Creating functionality with multi-phasic polymer-based soft matter
To integrate various functionalities in the materials of the future, only new chemistries will not be sufficient. Rather, formulating multi-phasic materials and engineering their microstructure will become even more essential than it already is at present. Also many biological, food and life science materials are multi-phasic mixtures consisting of liquid-liquid, solid-liquid, gas-liquid or even liquid-liquid-solid mixtures. To obtain a synergistic combination of the component properties, not only the material formulation but also the processing conditions need to be carefully designed. Therefore, our research aims at understanding the relations between the microstructure and resulting properties as well as the flow-induced development of these microstructures. Additive manufacturing provides ample unexplored opportunities to develop multifunctional materials by designing shape, composition and microstructure in3D. Hence, 3D printing strategies using a wide variety of techniques including selective laser sintering (SLS), fused deposition modelling (FDM),stereolithography (SLA), direct ink writing, etc. are exploited for generating functional multi-phasic polymer-based materials. Applications of functional polymer-based soft matter range from the area of electronic materials (e.g. polymer nanocomposite electromagnetic shields, polymer dielectrics for energy harvesting or wearable sensors) to biological, biomedical and food applications(e.g. printable hydrogels and food products). Though used for very different applications, the physical principles, flow behavior and microstructure-rheology correlations of these materials can show striking similarities.
Uncertainty quantification
Due to the microstructure in soft matter, its response to external stresses (i.e., its constitutive behavior) is complicated and difficult to predict. However, an understanding of this response is important in many industrial applications, for example during material processing. We apply the framework of uncertainty quantification (UQ) to the flow of soft matter. This is done by using a combination of simple flow experiments and simulations (see also [link to numerical methods here]). By using UQ, we can identify which constitutive models are most suitable given measurements and prior information. Moreover, UQ supplies us with certainty bounds of our flow predictions, which is crucial information for the design and optimization of industrial processes.
Leveraging morphology in soft smart materials
Soft matter offers ample opportunity for design of new material and new industrial techniques such as 4D printing. Soft colloidal particles, in particular, can be used as smart building blocks for responsive materials – their size and their ability to swell and deform in response to different stimuli enables their integration in emerging applications at the micro and nanoscales. While synthesis of long-sought colloidal architectures is possible, autonomous shape-dependent assemblies of multi-particle systems remains an engineering challenge. In the Processing and Performance group, we develop mesoscale models and dynamic particle-based simulations that provide the versatility demanded to study efficiently the dynamic nature of particle morphology in soft matter, which playa fateful role.
Multiphase soft materials
Multiphase soft materials, such as foams and emulsions, are ubiquitous in everyday life with applications in the food, biomedical, and energy industry. In the Processing and Performance group we use exquisite experimental techniques to study such materials at all relevant length scales. Phenomena that occur at the microscopic level of the thin film that separates two droplets or bubbles can be readily studied using the Dynamic Thin Film Balance (DTFB) technique. Microinterferometry combined with precise pressure control allows us to visualize the film and to study film dynamics under a wide range of hydrodynamic conditions.
Film drainage is usually the rate-determining step in coalescence. The outflow of liquid from the film is controlled by the magnitude of the interfacial stresses. In this respect, a direct assessment of the interfacial stresses is done using interfacial shear rheometry (Double-Wall-Ring) and tensiometry (Langmuir trough, pendant drop). A correlation of the measured interfacial properties with FEM simulations and the DTFB measurements has allowed to shed light into the complex phenomena that control coalescence (i.e. drainage, instabilities, rupture).
Soft matter exists in abundance in nature and inspire numerous industrial applications. In the Processing and Performance group, soft matter technology is approached experimentally, theoretically, and numerically. Soft matter covers a wide spectrum of materials and hierarchical microstructures; our group’s work reflects that by characterizing soft matter from microscopic-to-macroscopic length scales. Our group leverages this knowledge in emerging technologies such as food printing and 4D-printing.
Thermal fluctuations
Intrinsic to soft matter is that the strength of the interactions is comparable to the thermal energy kBT. This also implies that the thermal fluctuations can playa key role in the behavior of soft matter. The microscopic constituents of soft matter (e.g., small suspended particles or droplets, coarse-grained beads in a polymer) experience Brownian motion. While thermal fluctuations are most often not visible on the macroscopic scale because they are averaged out, one of our research directions is concerned with situations in which thermal fluctuations do come forward as a significant contribution, e.g., in microrheology,micro-/nanofluidics, filaments, and thin films. Efforts in this direction are currently focused on method development and numerical simulations in relation to fluctuating viscoelasticity.
Creating functionality with multi-phasic polymer-based soft matter
To integrate various functionalities in the materials of the future, only new chemistries will not be sufficient. Rather, formulating multi-phasic materials and engineering their microstructure will become even more essential than it already is at present. Also many biological, food and life science materials are multi-phasic mixtures consisting of liquid-liquid, solid-liquid, gas-liquid or even liquid-liquid-solid mixtures. To obtain a synergistic combination of the component properties, not only the material formulation but also the processing conditions need to be carefully designed. Therefore, our research aims at understanding the relations between the microstructure and resulting properties as well as the flow-induced development of these microstructures. Additive manufacturing provides ample unexplored opportunities to develop multifunctional materials by designing shape, composition and microstructure in3D. Hence, 3D printing strategies using a wide variety of techniques including selective laser sintering (SLS), fused deposition modelling (FDM),stereolithography (SLA), direct ink writing, etc. are exploited for generating functional multi-phasic polymer-based materials. Applications of functional polymer-based soft matter range from the area of electronic materials (e.g. polymer nanocomposite electromagnetic shields, polymer dielectrics for energy harvesting or wearable sensors) to biological, biomedical and food applications(e.g. printable hydrogels and food products). Though used for very different applications, the physical principles, flow behavior and microstructure-rheology correlations of these materials can show striking similarities.
Uncertainty quantification
Due to the microstructure in soft matter, its response to external stresses (i.e., its constitutive behavior) is complicated and difficult to predict. However, an understanding of this response is important in many industrial applications, for example during material processing. We apply the framework of uncertainty quantification (UQ) to the flow of soft matter. This is done by using a combination of simple flow experiments and simulations (see also [link to numerical methods here]). By using UQ, we can identify which constitutive models are most suitable given measurements and prior information. Moreover, UQ supplies us with certainty bounds of our flow predictions, which is crucial information for the design and optimization of industrial processes.
Leveraging morphology in soft smart materials
Soft matter offers ample opportunity for design of new material and new industrial techniques such as 4D printing. Soft colloidal particles, in particular, can be used as smart building blocks for responsive materials – their size and their ability to swell and deform in response to different stimuli enables their integration in emerging applications at the micro and nanoscales. While synthesis of long-sought colloidal architectures is possible, autonomous shape-dependent assemblies of multi-particle systems remains an engineering challenge. In the Processing and Performance group, we develop mesoscale models and dynamic particle-based simulations that provide the versatility demanded to study efficiently the dynamic nature of particle morphology in soft matter, which playa fateful role.
Multiphase soft materials
Multiphase soft materials, such as foams and emulsions, are ubiquitous in everyday life with applications in the food, biomedical, and energy industry. In the Processing and Performance group we use exquisite experimental techniques to study such materials at all relevant length scales. Phenomena that occur at the microscopic level of the thin film that separates two droplets or bubbles can be readily studied using the Dynamic Thin Film Balance (DTFB) technique. Microinterferometry combined with precise pressure control allows us to visualize the film and to study film dynamics under a wide range of hydrodynamic conditions.
Film drainage is usually the rate-determining step in coalescence. The outflow of liquid from the film is controlled by the magnitude of the interfacial stresses. In this respect, a direct assessment of the interfacial stresses is done using interfacial shear rheometry (Double-Wall-Ring) and tensiometry (Langmuir trough, pendant drop). A correlation of the measured interfacial properties with FEM simulations and the DTFB measurements has allowed to shed light into the complex phenomena that control coalescence (i.e. drainage, instabilities, rupture).