Robotic Winding
The research theme of incorporating winding structures in the construction industry has gained increasing interest in recent years due to its potential to enhance the design and construction of structural materials. Bio composite ropes, which are made from a combination of natural fibers and a synthetic binding agent, such as resin, are a key component of this field of study. When hardened using resin, these ropes are able to provide structural support and resist external loads, making them suitable for use in winding structures. In addition to the use of bio composite ropes, this field of research also involves the application of parametric design optimization techniques and graphical representations of force directions to define the winding geometry and minimize material usage. By investigating the possibilities of using winding structures in construction, researchers aim to discover new and innovative ways to improve the performance, efficiency, and sustainability of the built environment.
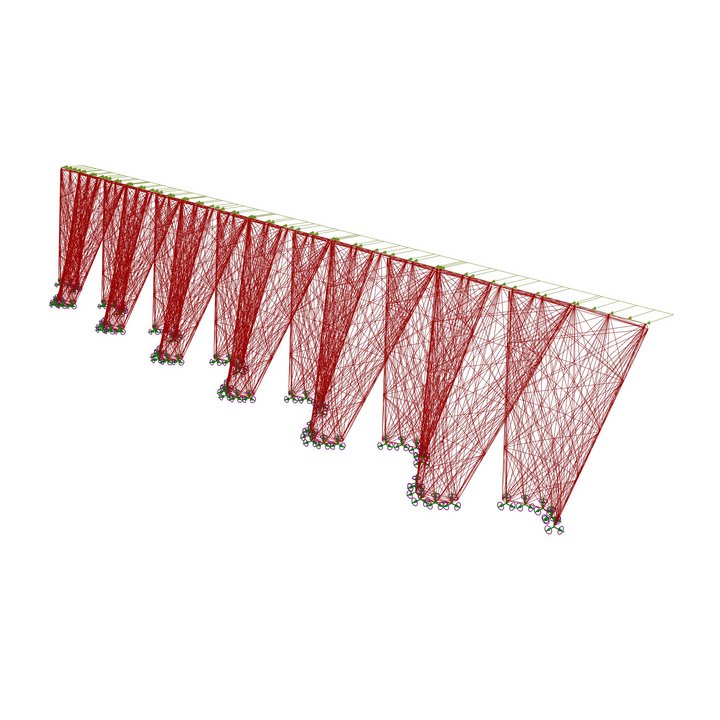
ROBOTIC FILAMENT WINDING OF A LIGHTWEIGHT AXIAL COMPRESSIVE ELEMENT
Lotte Krijnen
In this graduation project the aim is to design and manufacture a lightweight structural element to take axial compression, that will be manufactured through robotic filament winding out of biobased sisal rope. During the first phase of the project, a typology optimization and FEM-analysis were conducted to study the flow of forces for a hinged column loaded in axial compression. This resulted in a slightly convex-shaped column design that refers to the Euler buckling shape of a pinned column. A numerical model was set up in Rhino using a script written in Grasshopper and optimized for three fitness function values: to minimize mass and internal elastic energy, and to maximize resistance against buckling. An iterative sizing optimization was then conducted to distribute the material based on the strain energy, resulting in a final design with slightly decreased mass and increased stiffness and buckling resistance. A non-structural formwork was designed to wind the sisal rope around the optimized design, using an expanding mechanism that can be removed through the smaller end of the column after the fibre composite column is fully cured. The final structure was tested in an axial compressive experiment. A maximum compressive force of 21.4 kN could be measured. The governing failure mechanism of the column was local buckling. As the fiber composite columns are not one single element but an interaction of multiple elements, it seems more likely that local buckling of thesisal rope occurs before global buckling of the column.
Structural optimization of a bio-based bridge balustrade
Lizzy Louer
In this master's graduation thesis, the goal was to produce a bio-based bridge railing through numerical optimization and robotic winding. Many aspects are incorporated in this research, such as computational design, materialization, and product manufacturing. First of all, a preliminary design, together with the chosen production method of coreless filament winding, is used to investigate material possibilities. Secondly, the production method is established and thoroughly tested. The structure is finalized with a discrete ground structure optimization. Through smart material distribution, this results in the most lightweight structure within the material and production limits. A robot path is scripted from the extracted optimization geometry to manufacture the final structure. This structure consists of two wound panels, which are tested with a horizontal load at the top edge, mimicking the load of people leaning against the bridge railing. This research displays a construction method with bio-based materials and one of its possibilities. The success of a functional bridge railing structure is a huge achievement and a great step forward in this innovative field.
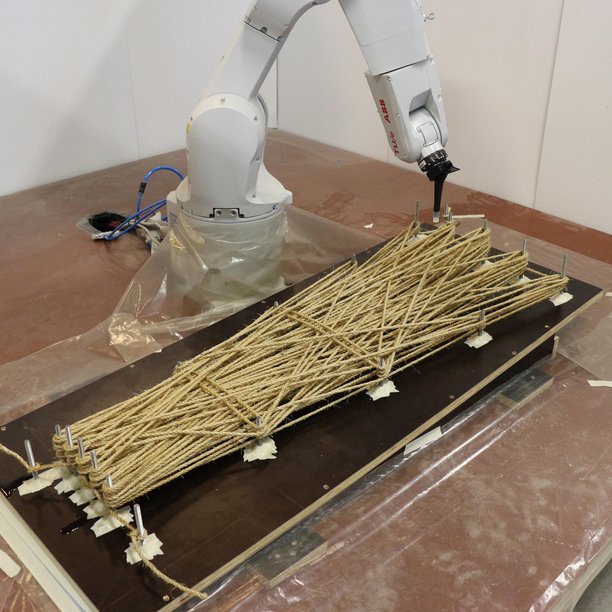
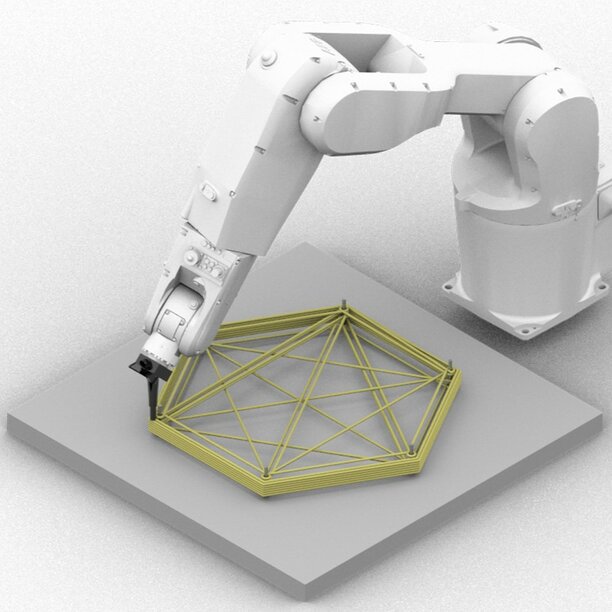
Robotic Winding
Daniƫl Jansen
Nature has highly efficienct structures from which we can learn. She thickens areas of high stress and leaves areas of low stress thin, all without a blueprint, but as a direct effect of the forces flowing through the material. There is an intrinsic efficiency in the way nature does this; she has essentially been running optimization processes for millions of years. The material of choice in nature is often fibers, which are versatile due to their strength and modularity. To emulate this observed efficiency in manmade structures, there are challenges to consider. The first is design. Computer models could approach optimal design using mathematical methods, similar to the way optimization works in nature. The design is iterative and adjusts to changing flow of force by displacing the material towards high stresses. Another challenge is material placement. Nature distributes material using complex biological systems, whereas current building methods tend to make structures as generalized as possible. However, using computer-aided design, high complexity can be achieved and directly fed into a robotic manufacturing process. The goal of this research is to apply these principles to create a panel-based, fully integrated construction method.